Mephedrone is predicted to have stimulant effects akin to amphetamines based on its chemical makeup. Mephedrone has been shown to act as a substrate for monoamine transporters, which causes a depolarizing current to be mediated by the transporters and releases monoamines via reverse transport. Mephedrone given to rats increases extracellular dopamine (DA) and serotonin (5-HT) in the rat brain, comparable to the effects of MDMA, according to several studies.
These findings showed that this substance interacts with DA and 5-HT transporters in a manner resembling those of other amphetamine compounds. Mephedrone users showed signs of wanting to redose after taking the drug once, which led them to take greater doses. This pattern of usage suggests a possible overdose risk.
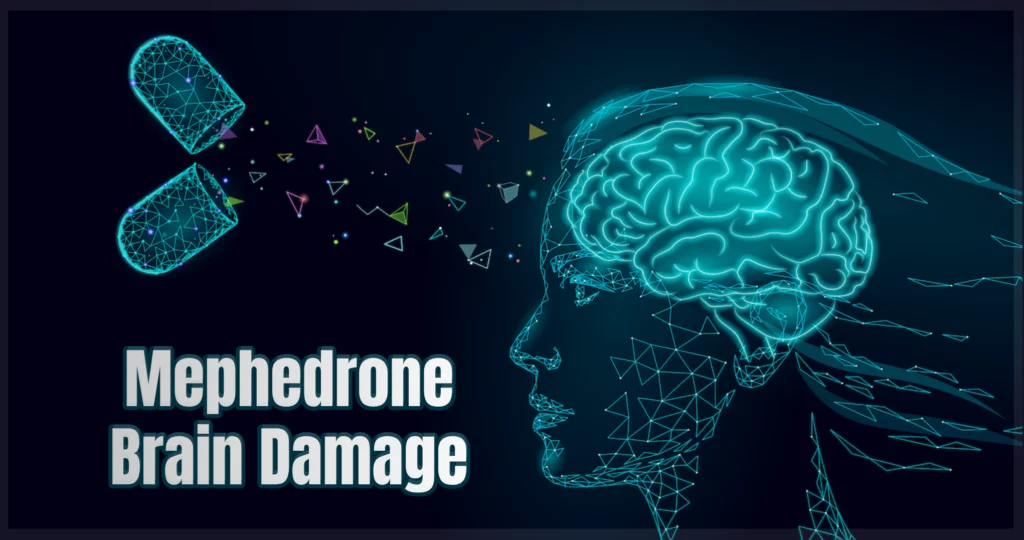
Acute adverse effects of MDMA and beta-ketoamphetamine intake in humans frequently include agitation, psychosis, tachycardia, and hyperthermia. Such symptoms force amphetamines and cathinones users to seek medical attention. A persistent practice of using this chemical may potentially have more modest long-term impacts on brain damage (Mephedrone brain damage). There are contradictions regarding its neurotoxicity in rodents, though.
Mephedrone could have a species-dependent neurotoxicity, similar to MDMA. Then, it’s crucial to compare the results while keeping the species that the studies were done on in mind. To yet, researchers have discovered that giving mephedrone to mice does not harm their DA or 5-HT systems. Nevertheless, the studies did not extent the evaluation of DA to brain areas other than striatum or were performed with a drug exposure schedule not adjusted to mephedrone pharmacokinetics.
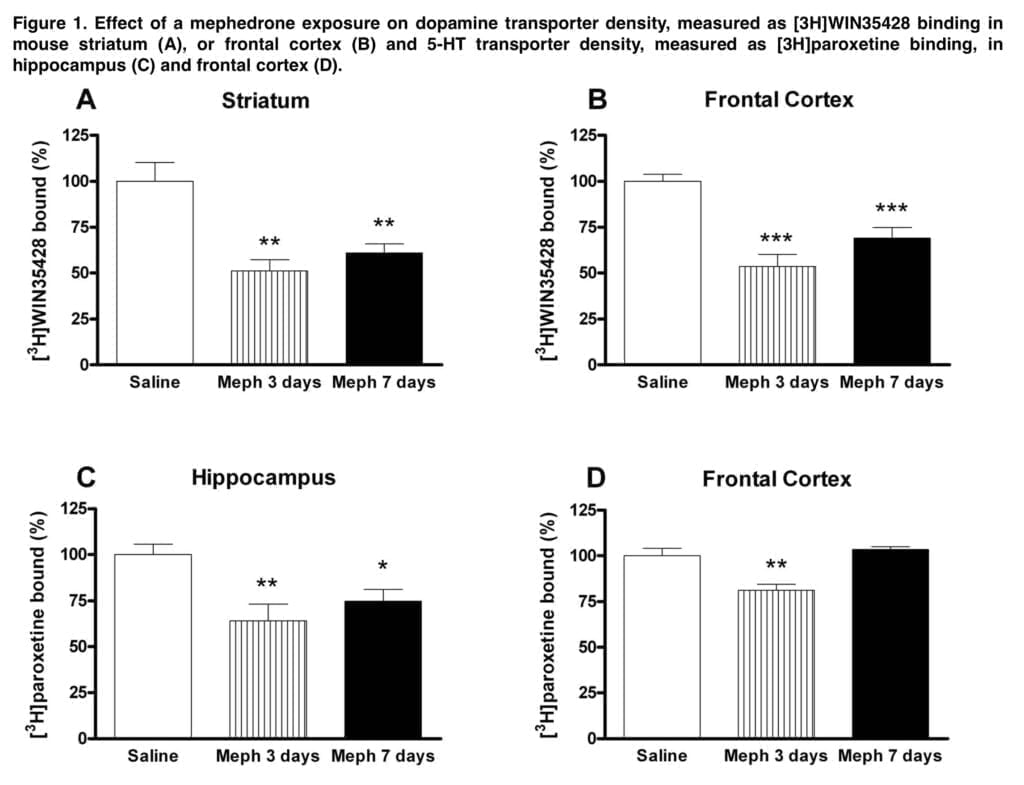
Mephedrone Brain Damage: about study
This study sought to fill certain gaps in the literature by looking at the neurotoxicity profile of mephedrone in mice. The majority of writers discussed MDMA’s neurotoxic effects seven days after exposure and methamphetamine’s neurotoxic effects three days after exposure. We examined the neurotoxic injury induced by mephedrone at 3 and 7 days after finishing the exposition.
It is crucial to get as much mechanistic data as possible on mephedrone’s actions and neurotoxic consequences. In this context, we have assessed the in vivo effects of this cathinone using a variety of dosing regimens, complemented by in vitro research. The severity of the effects of MDMA abuse is stated as being greatly influenced by the size of the initial hyperthermic reaction.
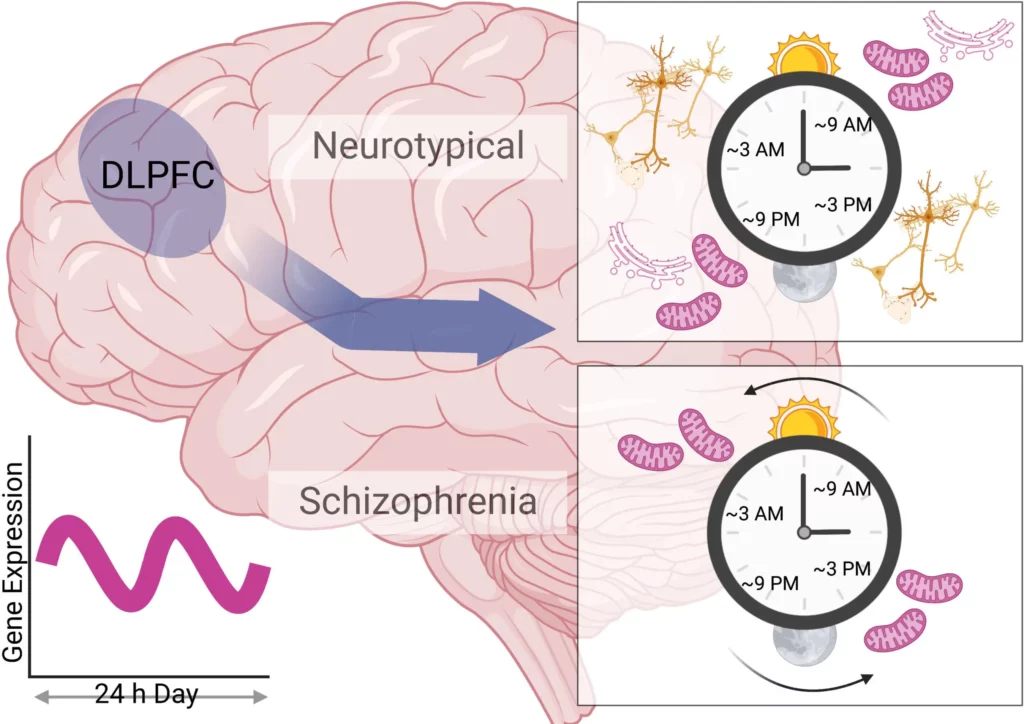
This means that taking the drug in a hot, packed dance club enhances the likelihood of future brain neurotoxic effects. The neurotoxicity experiments with amphetamine derivatives are typically carried out at increased ambient temperatures to imitate these typical circumstances of drug exposure. As a result, high room temperatures were used for the current investigations. Previous articles that were published did not take this circumstance into account.
Adolescent mice were utilized in the current study, a characteristic that is similar to that of young adult consumers. We show that mephedrone causes damage to nerve terminals in the frontal brain when used on a schedule that simulates human “weekend usage“.
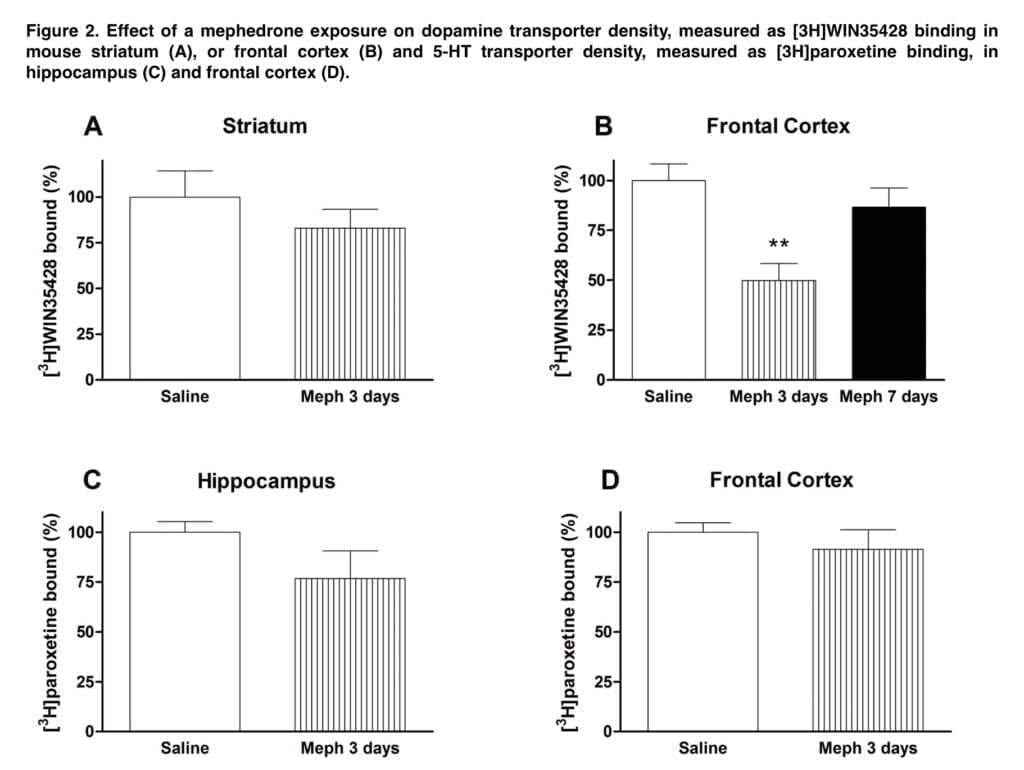
Experimental Procedures
The following substances were used in the present study: mephedrone hydrochloride, [3H]ketanserin, paroxetine, [3H]raclopride and WIN35428. Considering that typical doses of mephedrone consumed in an evening/night are approximately 500 to 1000 mg, in our study we used a dose of 25 mg/kg, which corresponds to a dose of 2 mg/kg for an adult.
The two-hour interval between doses was chosen according to the half-life of mephedrone. The drug was administered subcutaneously to mice according to three different regimens: the first regimen was to administer four doses of saline or mephedrone at a dose of 50 mg/kg two hours apart; the second regimen included a dose of mephedrone 25 mg/kg; the third regimen was to administer three doses of saline or mephedrone 25 mg/kg at two-hour intervals over a period of two days.
A lubricated, flexible rectal probe coupled to a digital thermometer was inserted into the rectum (1.5 cm) 45 minutes after the previous dosage to measure the rectal temperatures. 40 seconds after the probe was inserted, the rectal temperature was recorded. We carried out one more experiment. Some animals’ brains were split into its two hemispheres after being severed from their heads. We used cellular fractionation in one of them to produce a fraction rich in endosomes and another with a high plasma membrane composition.
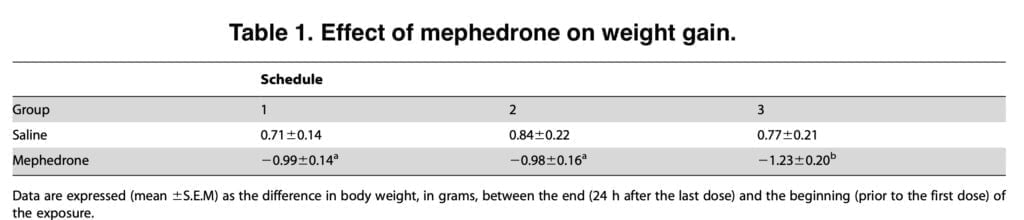
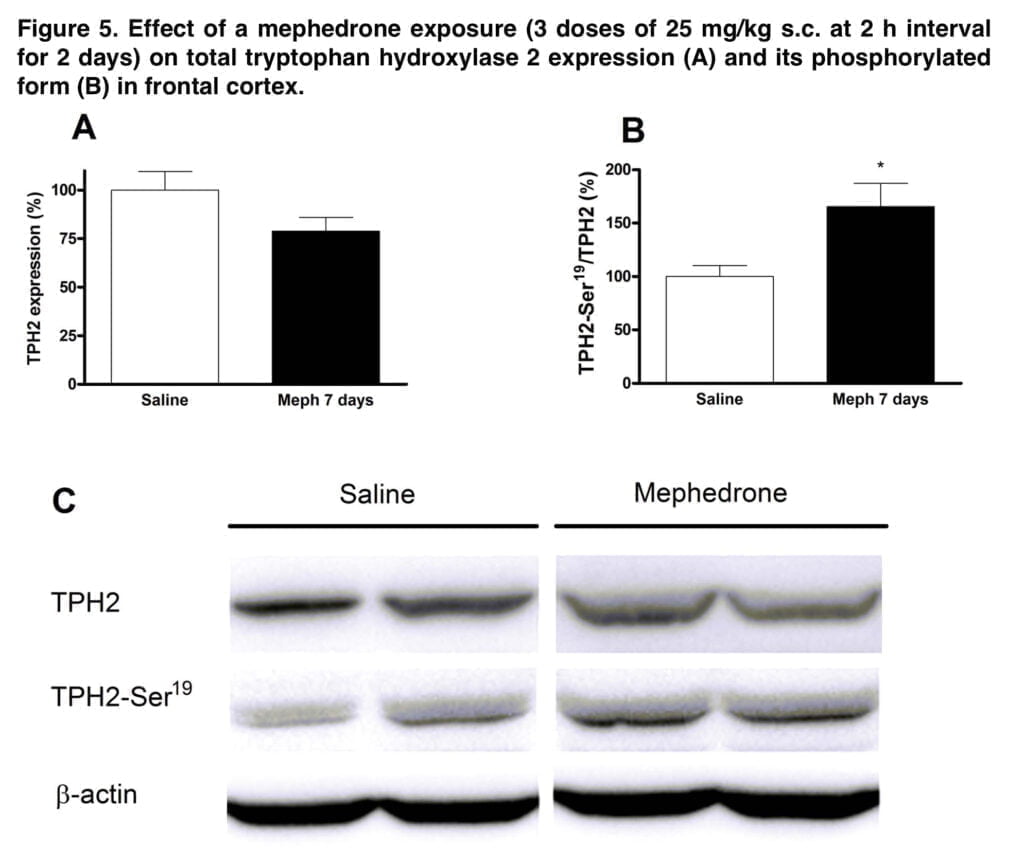
Using WIN35428 binding experiments, the density of the DA transporter in the membranes of the striatal or frontal cortex was determined. These were carried out in tubes containing, respectively, 50 or 100 mg of membranes and 250 or 500 ml of 5 nM WIN35428 in phosphate-buffer. In the presence of 30 mM bupropion, the incubation was carried out for 2 hours in order to evaluate non-specific binding. Clomipramine (100 mM) was used to determine non-specific binding. After 150 mg of protein were incubated at 25 uC for two hours in a Tris-HCl solution, the specific binding of 0.05 nM [3H]paroxetine was measured to determine the density of the 5-HT transporter in the membranes of the hippocampus and frontal cortex.
Tyrosine hydroxylase (TH) and tryptophan hydroxylase 2 (TPH2) levels were determined using a conventional western blotting and immunodetection methodology. PVDF membranes were blocked overnight with 5% defatted milk in Tris- buffer and incubated for 2 h at room temperature with a primary mouse monoclonal antibody against TH or with a primary rabbit polyclonal antibody against TPH2 and anti-(phosphor-Ser-19)TPH2.
Software was used to evaluate scanned blots, and dot densities were represented as a share of those from the control. In order to correct variations caused by protein content, beta-actin immunodetection (mouse monoclonal antibody, 1:2500) was utilized as a control of load uniformity for each lane.
The following technique was used for immunohistochemical analysis: Animals were given sodium pentobarbital to induce anesthesia before having 4% paraformaldehyde in phosphate buffer injected into their hearts. Brains were extracted, post-fixed for two hours in the same solution, then cryoprotected for twenty-four hours in a 30% sucrose/phosphate buffer.
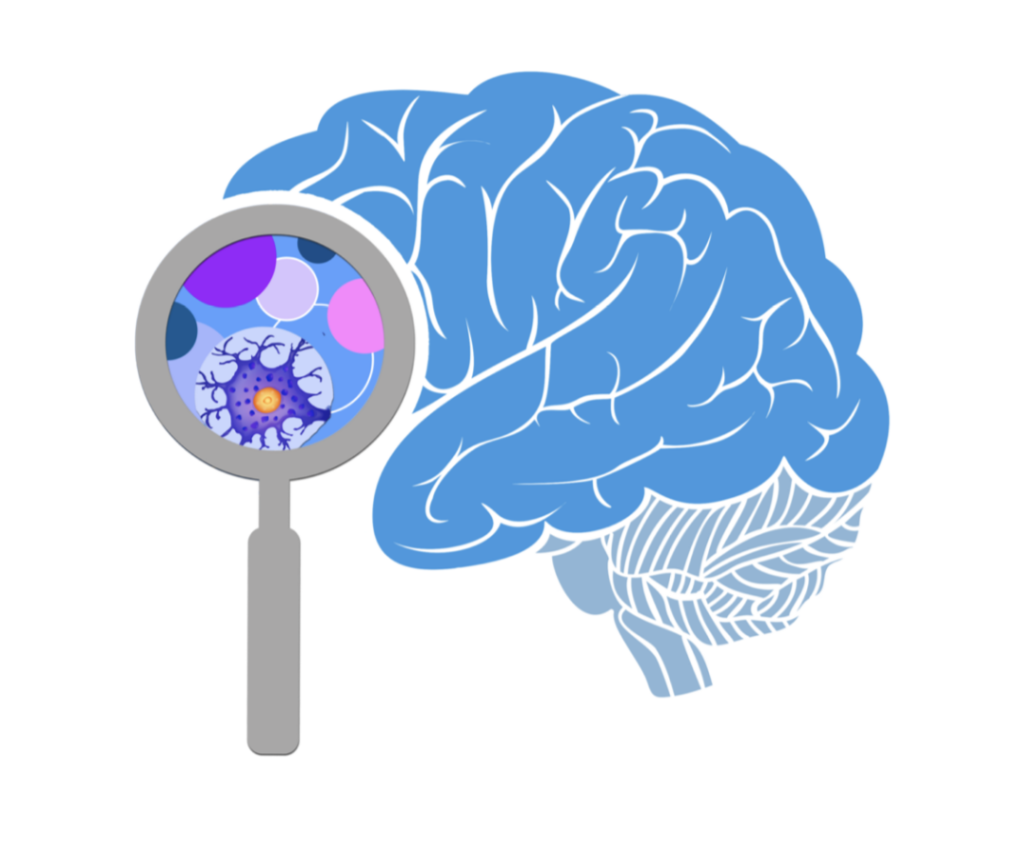
Afterwards sections were incubated with avidin-biotin-peroxidase complex. Peroxidase reaction was developed with diaminobenzidine in phosphate buffer and 0.02% H2O2, and immunoreacted sections were mounted on gelatinized slides. Stained sections were examined under a light microscope.
Primary neuronal cultures of cerebral cortex were obtained from mouse embryos. The cerebral cortex was dissected, meninges were removed, and tissue was incubated for 20 min in trypsin. Dissociated cells were washed with phosphate buffer containing 0.6% glucose and centrifuged for 5 min.
The cells were redissociated in Neurobasal medium with L-glutamine, sodium bicarbonate and penicillin and streptomycin, containing B27 supplement and 10% horse serum. After four days, the culture medium was changed to Neurobasal medium containing B27 and no antioxidants. The concentration of HS was reduced in half prior to treating the cells. After 8–9 days in vitro with various mephedrone doses and exposure periods, the cells were employed for research.
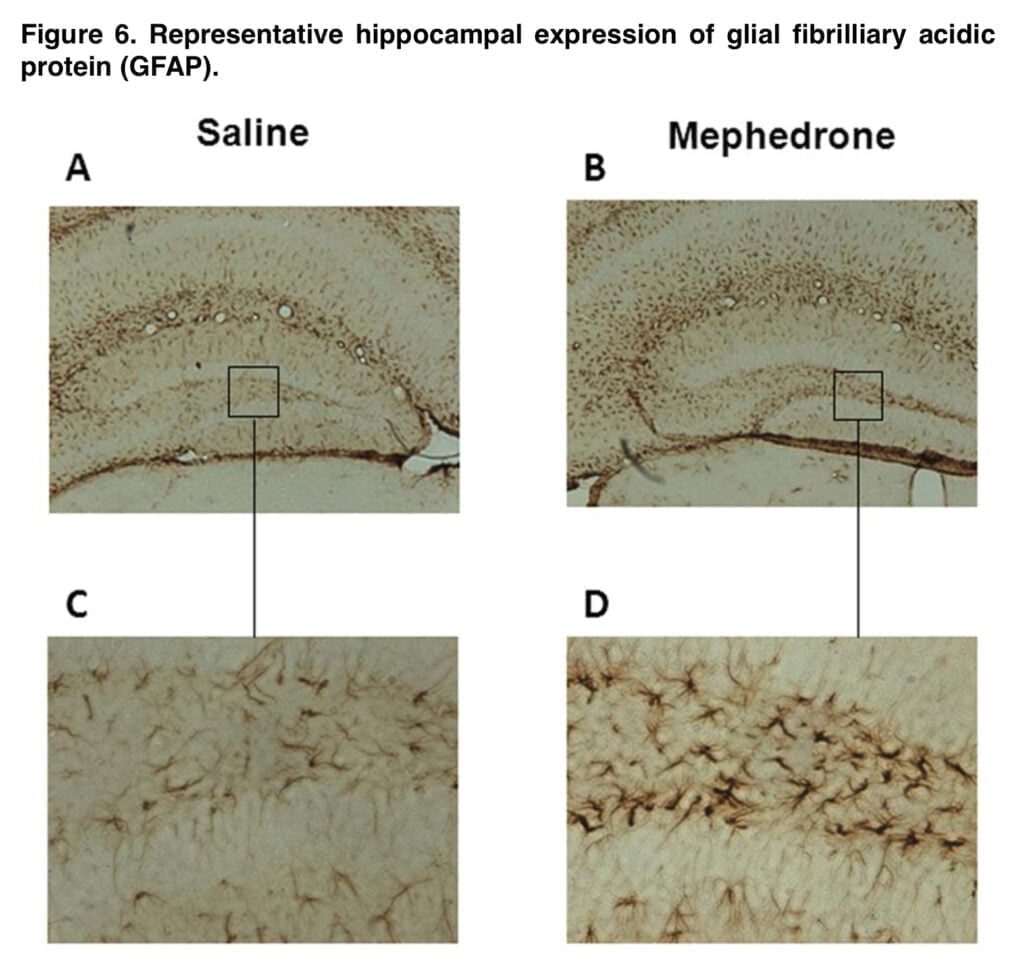
Analysis and discussion of the results
Cathinones’ simple accessibility and initial position as legal highs may have led to their rising use as recreational drugs. The effects of cathinones on long-term users are still unknown due to the relatively recent history of their usage as recreational drugs. It was assumed that mephedrone would cause neuronal damage due to its structural resemblance to well-known neurotoxic psychostimulants like methamphetamine and MDMA.
However, there are significant inconsistencies when it comes to the neurotoxicity brought on by cathinones. Different species, dosages, routes of administration, or environmental temperatures might all be to blame for the variable findings. Recently, the media has reported on some instances of violent behavior, and even cannibalism, as a result of exposure to new designer medications. These incidents, however, have not been well recorded.
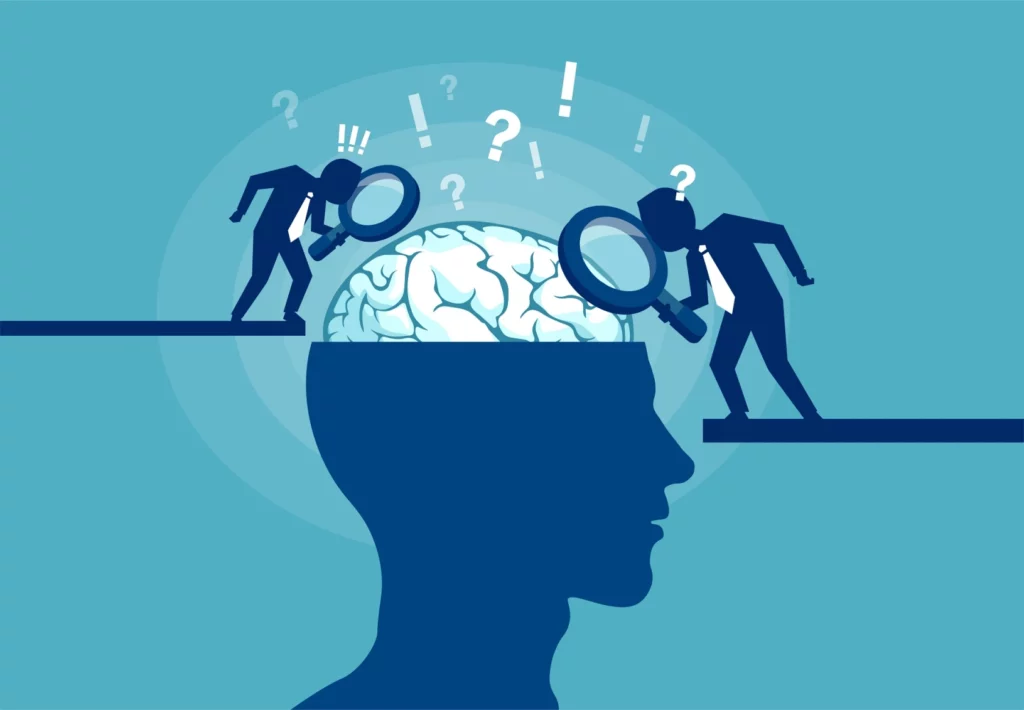
All mephedrone regimens used in this study produced initial stereotypy, which manifested as frequent self-licking, which was then followed by aggressive behavior that resulted in self-injuries. This is an especially important factor to be taken into account, seeing as it required animals to be housed individually. Further studies are needed to determine the mechanism implicated in this feature induced by mephedrone and its relationship with the exposed dose.
A common acute adverse effect of ingesting beta-ketoamphetamine and amphetamines in humans is hyperthermia. Cathinone exposure in rodents results in considerable core temperature rises as well. The temperature has been examined during administration of multiple doses of the drug. The amplitude and direction of the hyperthermic reaction seen in the current trials may be influenced by the animal strain, dosage, and ambient temperature.
The current data show that mephedrone reduced the thermoregulatory response at high ambient temperatures; this impact remained during the two day exposure, despite the fact that lethal hyperthermia was not detected at the evaluated doses in mice. However, more study is needed to determine if hyperthermia plays a complimentary or crucial role in the development of mephedrone-induced neurotoxicity.
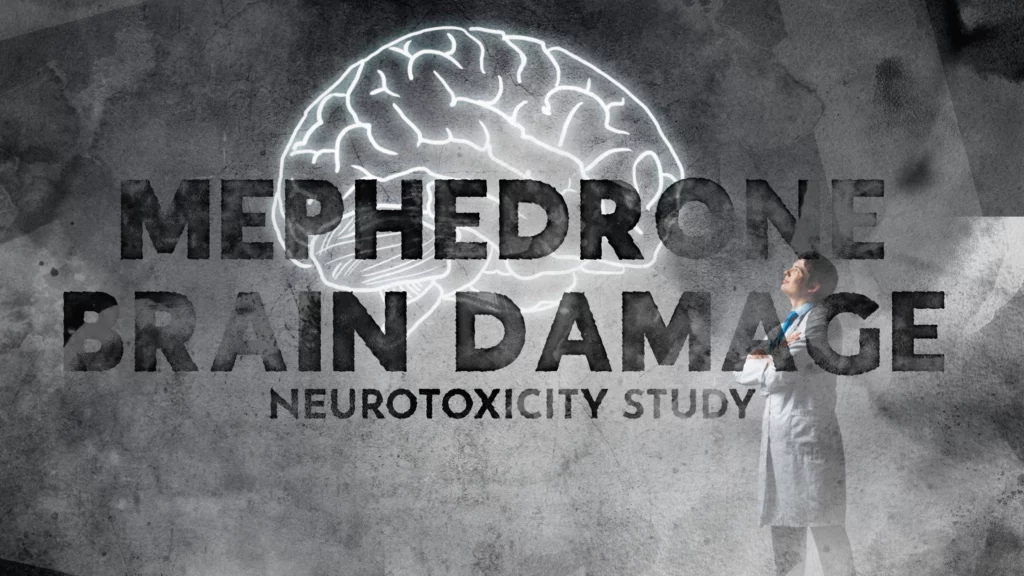
We thought it suitable to imitate the common behaviors of “stacking” (taking numerous doses at once in order to improve the intended impact and/or overcome tolerance from earlier usage) and “boosting” in our attempt to represent recreational mephedrone use. This is why we decided to provide mephedrone in numerous dosages on each day of the exposition. In schedule 3 we repeated the exposition the next day, simulating a pattern of a recreational weekend use.
The crude membrane preparation used in the current experiments was confirmed to collect both the synaptosomal membrane and the endosomal fraction, so it is important to keep in mind that it is impossible to determine whether or not a transporter redistribution occurred when there was no decrease in radioligand binding in these preparations.
The crude membrane preparation used in the current experiments was confirmed to collect both the synaptosomal membrane and the endosomal fraction, so it is important to keep in mind that it is impossible to determine whether or not a transporter redistribution occurred when there was no decrease in radioligand binding in these preparations. We show that mephedrone caused a substantial drop in DA transporter density in mouse striatum and frontal cortical membranes that sustained 7 days after exposure, provided at dosages (i.e. schedule 1) that approximate a high exposure in people (about 1.5 g/session).
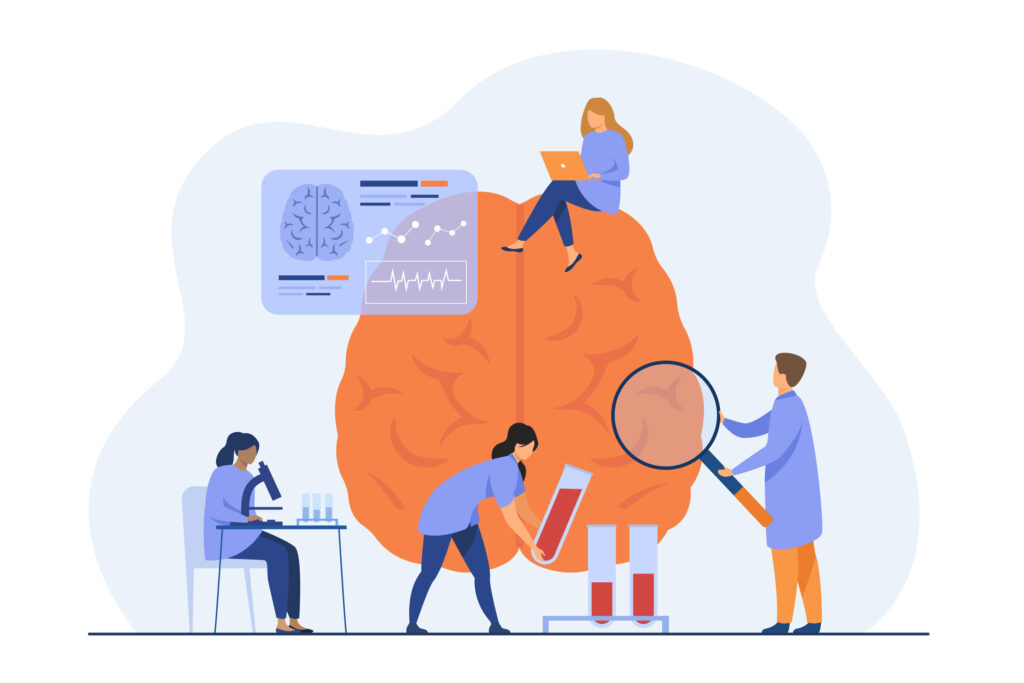
This effect was accompanied by a significant loss of 5-HT transporters in the hippocampus. However, at these high doses, acute cardiovascular toxicity of mephedrone is likely and probably outweighs neurotoxic effects. Lower doses (25 mg/kg) were used in the following investigations. Only a brief drop in cortical DA transporter was caused by schedule 2 dose schedule, indicating a transitory regulatory impact. Moreover, neither the frontal brain nor the hippocampus showed any discernible loss of 5-HT transporter.
The schedule that most closely resembles the average weekend consumption pattern is Schedule 3 (three doses of 25 mg/kg for two consecutive days). Mephedrone caused loss of DA and 5-HT transporters after this exposure, which was particularly noticeable in the frontal brain and the hippocampus, respectively.
This schedule also resulted in a substantial decline in each enzymatic marker, which was associated with the reduction in radioligand binding, contributing to the monoamine deficit. The initial and rate-limiting steps in the production of DA and 5-HT are respectively catalyzed by TH and TPH. In the brain, 5-HT is synthesized by the isoform TPH2. The rise in Ser-19TPH2 in mephedrone-treated mice appears to indicate a compensatory mechanism in the uninjured 5-HT terminals. The decrease in transporter binding and enzyme levels, along with astrogliosis, hint to an insult at the nerve endings.
After the third mephedrone regimen, we looked at how the density of these receptors changed in the striatum. Both 3 and 7 days after mephedrone exposure, the density of D2 receptors remained below control levels, suggesting that these animals may be more susceptible to drug addiction. Additionally, 3 days after exposure, we discovered a large transitory drop in the number of 5-HT2A receptors, which may have been a neuroadaptive reaction to the rise in 5-HT release brought on by mephedrone.
These findings concur with those made public by Scheffel, who showed how repeatedly administering MDMA produces a temporary down-regulation of 5-HT2 receptors, which are mostly numerous in the frontal brain. In order to evaluate a potential concentration that may be harmful to neuron survival, which is known to be fairly high for most amphetamines, we conducted with vitro tests in cortical grown cells. We discovered that mephedrone has a cytotoxic impact on these cells that is concentration-dependent and dramatically rises with exposure time.
It must be noted that in primary cultures of hippocampus neurons, this cytotoxicity is greater than that of MDMA. Our prior research on the pharmacokinetics of mephedrone in rats indicates that a dosage of 10 mg/kg administered intravenously results in an extrapolated blood concentration of mephedrone of roughly 5.6 mM. Then, a dose of 25 mg/kg would correspond to a mephedrone concentration of about 14 mM that is about 15 times lower than the LD50 in cultured cortical cells.
Conclusion
Because there were no indications of striatal or cortical astroglial activation in the mephedrone-exposed (25 mg/kg) mice in the current investigation, it is crucial to stress that no neuronal loss is suspected. The dentate gyrus only showed a few reactive astrocytes. We also didn’t see any microglial activation (data not shown). Because neuronal death is acquired at greater concentrations than those found after in vivo exposure, results from cortical cultivated cells appear to trend in the same manner.
The in vivo metabolic route of mephedrone is comparable to that of MDMA, thus we cannot rule out the potential that some of its metabolites may have harmful consequences of this kind. Finally, our findings show that mephedrone exposure schedule affects mephedrone-induced brain damage differently (dose, number of administrations and intervals). Mephedrone causes neuronal damage, which becomes evident when taken at 2-hour intervals.
This is consistent with our earlier study that described the pharmacokinetics of mephedrone. We discovered a loss of frontal cortex dopaminergic and hippocampus serotoninergic neuronal markers together with astrogliosis using this dosage interval and repeated doses for two days under a higher ambient temperature, indicating the existence of damage at nerve terminals. This process is accompanied by a compensatory mechanism that involves a rise in the phosphorylated form of TPH2, a change in DA transporter subcellular distribution, and a decrease in D2 receptors.